Convection is something you probably deal with every day as a pilot, whether you’re aware of it or not. When you hear the word “convection” you might be thinking of a cumulus cloud or maybe the lively bubbling in a pot of boiling pasta. But the exact definition of convection is the spontaneous release of kinetic energy that occurs when a buoyant condition is created—in our case, in the atmosphere. Convection is one of the most common ways in which thermal energy is transferred.
Thermal Transfer
To understand this better, let’s go on top of an imaginary house with a chimney, and try to take measurements to see what the fireplace is doing. We mount a thermometer to the top of the chimney. If the mercury moves because the heat has spread through the materials and up the chimney, heating the thermometer through its attachment points, this is known as conduction.
If the thermometer registers an increase from being directly illuminated by an exceptionally hot and dazzling fire, this is radiation, much of which is in the infrared part of the spectrum. If a fan blows air up the chimney onto the thermometer that registers the heat, this is advection. And finally, if the heat rises through the chimney on its own, complete with the soot and smoke, this is convection, caused entirely by the buoyant warm air rising. These four methods are all of the key methods of heat transfer we work with in meteorology! Simple enough, right?
As you might guess, all of these processes are interconnected. The typical thunderstorm on a hot day is the manifestation of convection due to the sun shining on the hot ground. This in turn was caused by radiation, due to the ground being heated from short-wave radiation from the sun.
But we don’t always need sunshine. Advection due to winds blowing in from someplace warm can help infuse the air with buoyancy and produce the storms. This is a common cold-season process. And conduction is another method. If you were one of those in Buffalo who suffered damage from the lake effect snows last winter, the conduction of heat into the air mass from Lake Erie was the root cause of your misfortune, and that heat in turn came from many months of radiation, conduction, and advection working on the lake temperatures.
I will leave the topics of conduction, advection, and radiation as a separate subject in my forecast books. But convection has immediate effects on all aspects of flight, ranging from trivial issues like your comfort to serious problems like low IMC and exceeding structural limits. For that reason it pays to get to know the subject a little better.
Buoyancy and Motion
As we mentioned, convection is caused by buoyancy. The atmosphere is a fluid, much like the ocean, so parcels (imaginary boxes) that are less dense than their surroundings tend to float. What type of wood is more likely to float: balsa wood or ebony? You don’t have to think too much about this because balsa is lightweight and has a high ratio of size to weight. It’s not a dense material, so it readily floats when placed in water. Ebony of course is quite dense and heavy per unit volume, so it sinks right down like a rock, straight to Davy Jones’s locker. This is because its density exceeds that of water.
Air is not the same as wood of course, but things work the same way because air has a certain density. This is based mostly on its gases, which are constant, along with the temperature and amount of water vapor. Temperature is by far the biggest contributor to density. Warm air has low density, while cold air has higher density. Moisture has an influence too but only about 10 percent that of temperature. Because of that, we won’t worry about moisture in this article, but for the record, moist air contributes to low density in a parcel.
All of this is important because buoyancy is closely correlated with the vertical velocity of a parcel. For every one degree C increase in temperature, a parcel accelerates by 0.003 g, giving a vertical velocity of 4 knots after one minute. Obviously it does not take much heat at all to get the atmosphere churning and start bumping your airplane around.
Stability Sets the Stage
The atmosphere has a significant effect on buoyancy because it can work to either suppress or amplify vertical accelerations. This quality is known as stability. It’s determined by the lapse rate: the change in the atmospheric temperature with height. All you need to do is determine whether the atmosphere best represents a warm-over-cold situation or cold-over-warm.
There is a little bit of leeway with the warm vs. cold terminology, so don’t take it too literally. This is because of a complicating factor: the decrease of pressure with height and the resulting effect of adiabatic heating and cooling. A strong decrease in temperature with height is always “cold over warm.” But a temperature increase with height, an isothermal layer, or even weak cooling with height is sufficient to qualify as “warm over cold.” That’s the only confusing thing you will need to worry about. So with that settled, let’s continue.
So let’s first look at a warm-over-cold situation. Remember that slight cooling qualifies. But if the temperature increases with height, this is an inversion. You undoubtedly have heard this term and its relationship with smog, haze, and poor air quality.
Either way, a warm-over-cold layer is considered a stable layer. The weaker the lapse rate, the greater the potential for haze, fog, stratus, and stagnation of the air mass as seen in the photo on the opposite page. Such air is not conducive to buoyancy because air that rises through this layer soon ascends into a region with a lot of surrounding warmth. Since these surroundings already have low density, the parcel decelerates and is forced to sink, much in the same way that an overloaded Cessna trying to take off at Jackson Hole on a hot summer day struggles to gain altitude.
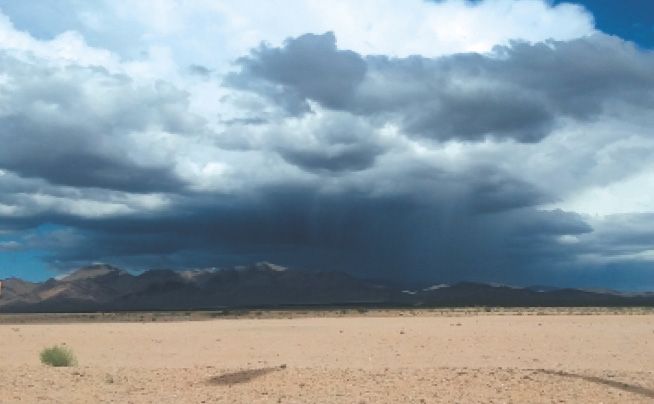
The other situation is cold-over-warm, in which temperature cools strongly with height. We refer to this as an unstable layer, which also has a steep lapse rate. Air that rises into this layer accelerates because the surroundings progressively become much cooler relative to the rising parcel, and this amplifies the effect of buoyancy. Cumuliform clouds predominate, and fog and haze tend to be dispersed, especially if prevailing winds are not light and variable.
In meteorology stable is simply that. But with unstable layers, we deal with two types: conditional and absolute.
Absolute instability is an extreme form of a cold-over-warm layer, usually caused by extremely hot conditions at the bottom of the layer. Nearly all of it occurs close to the ground whenever there’s strong surface heating. The lapse rate is so strong that the air must overturn immediately, and is set into motion until the buoyancy can be dispersed. When you look down the runway and see shimmer on a hot day, that’s the absolutely unstable surface layer overturning in the presence of strong heating. Thermals form from the effects of this heating.
Conditional instability is a weaker form of cold-over-warm and makes up the majority of unstable situations we see on weather charts. This type of atmosphere gets its name “conditional” because vertical motion is suppressed if saturation has not occurred, but it actually amplifies it if the parcel is saturated. This is due to the contribution of latent heat from condensation. A good example is when you’re watching a thunderstorm from a distance. There’s no thunderstorm at your location because the air has not undergone enough lifting overhead to saturate and release latent heat. But elsewhere, where air has been forced upward due to a front or a mountain range, air parcels easily rise into large thunderstorms. (See photo above.)
Turbulence
Turbulence describes the forces that act on a plane due to large differences in motion over small distances. These distances are on the scale of centimeters and meters. Most turbulence is vertical, of course, but we see horizontal changes as gusts. This is why gusts reported in a METAR observation are an excellent clue that suggests both instability and low-level turbulence.
Not surprisingly, convection is a major source of turbulence. Convection emerges from the contact layer at the earth’s surface (or less frequently, from moist elevated layers), and rises as thermals in a single plume or breaks up into numerous eddies. Other motion is from sinking within the surroundings, as convective cells develop across a given area, as well as from mixing of air from different altitudes.

So why are some atmospheres turbulent and some not? As you might guess, the background instability is a key factor. When there’s strong surface heating or strong cooling aloft, convection will occur with even faster accelerations, producing large vertical velocity differentials over a short distance. This is more likely to result in moderate to severe turbulence. Instability indicators like lapse rate, lifted index, CAPE, and vertical totals are useful tools for assessing turbulence potential.
Of course we can’t forget the effects of small-scale, pre-existing disturbances in the atmosphere. Some common sources are mountain waves and vertical wind shear. While these don’t produce convection by themselves, unstable environments with strong lapse rates help amplify small-scale disturbances. So when there’s strong heating of the surface or strong cooling aloft, high winds acting on an area can more readily convert into clear air turbulence and mechanical turbulence. Powerful cold-weather systems crossing the Rockies often have a combination of both cold-air advection aloft and strong heating below in the warm sector, which doubles the instability. The clear areas behind cold fronts underneath the upper-level trough are especially favored for low-level turbulence.
This turbulent flow may also produce enough mixing and instability to raise dust in some parts of the Southwest states, especially between the Sierras and the Mojave Desert and the western Great Plains. This dust is usually picked up by gusty winds and eddies, and lofted skyward with deep thermals in the highly unstable low-level layers near these systems.
A Few Final Techniques
As we pointed out earlier, stable layers are associated with fog, haze, stratus, and horizontal dispersion of smoke. Unstable layers support extensive vertical motion, so fog, haze, and stratus are all quickly dispersed, and smoke rises vertically. Because of the diffusion of haze, unstable layers may offer remarkable visibility, sometimes with clear blue skies. This is not true when there’s tropical moisture around, but in the dry unstable air behind a fresh cold front (cold air mass over warm ground) these are the days when you can see that distant mountain 80 miles away.
Be aware that stable and unstable layers are bound within specific layers, and there may be a mix of these in the environment. A good example is on the Great Plains during a stormy day. From the surface to 5000 feet MSL the atmosphere will often be unstable, allowing for extensive cumulus development. But a capping inversion is often found between 5000 and 10,000 feet MSL due to southwest mid-level winds bringing in warm, dry air from the Southwest Desert and the Mexican plateau. And from 10,000 to 40,000 feet we find a layer of very steep lapse rates, produced by strong dynamic lift.
The result is a lot of cumulus that rise and spread out beneath the inversion, producing stratocumulus layers at 5000 feet. If lift is strong enough, this unstable layer may bust through the cap, coupling the lower layers with the unstable upper layer, resulting in severe thunderstorms.
Another example is quiet summer weather in the Gulf Coast region. From the surface to 10,000 feet we find mildly unstable air, allowing lots of cumulus to develop. But from 10,000 feet to the stratosphere we might find relatively warm air due to the presence of a subtropical high. The result is cumulus clouds that rise aggressively during the morning but spread out and dissipate during the afternoon.
The best way by far of assessing stable and unstable layers is using a sounding, or Skew-T. That’s beyond the scope of this article, and it’s not necessary for planning a flight. We covered the Skew-T in some detail in previous articles (January 2022 and March 2020). But the details we explored today are enough to give you a working understanding of what the sky is doing on any given day when you slip the surly bonds.
The effects of lakes and nearby oceans are worth mentioning because these vary according to the season, which in turn determines if the water is colder or warmer than the landmasses. During the summer, most lakes and oceans are relatively cool. Thermals from nearby landmasses rise and spread out, and are replaced from below by lake breezes moving inland. A sinking cell develops over the lakes to compensate.
During the wintertime, lakes and coastal waters tend to be warmer than their surroundings, especially if cold air is invading. This results in thermals over the water and the production of lake-effect convection downstream. This usually is made up of stratocumulus layers, but can grow into cumulus and cumulonimbus. Offshore stratocumulus is quite common during the cold season as illustrated above.
It’s helpful to be aware of topography and winds at your favorite airports and understand the patterns during different seasons. A brisk flow off Lake Michigan into Chicago always means good weather in June, but in December count on clouds, snow showers, and possibly IMC. If that December flow is unusually cold, the chance of bad weather is significant.
Tim Vasquez served as an Air Force meteorologist and recently was mission forecaster for the D-Day 50th anniversary B-1B sortie over the English Channel. The cloud cover forecasts were shaped mostly by instability, wind, and water temperatures, which are the same issues military forecasters struggled with in June 1944.