There’s an old Buddhist saying that the fool sees only the form, while the enlightened person sees the essence. It’s strange to think that religion could give us any insight into aviation weather. But in the same way that gods tend to be elusive, many of the important details in the atmosphere are unseeable and unknowable, and can only be inferred by balloon soundings, radar samples, and satellite measurements. This makes meteorology unusual—it is both a science and an art.
When you receive a forecast, you’re mostly focused on the values that are presented. It’s a cool day in November, and you’re at an FBO in Wichita, reviewing a planning chart, and see that winds at 25,000 feet will be from 220 at 80 knots. You listen to the ATIS and hear that winds are light easterly and the ceiling is broken at 3000 feet. Using just these values you have basic measurements for your journey. But the underlying form of the atmosphere lies past these numbers. I’ll show you how to tease those values out of commonly reported aviation weather values and understand what’s really going on.
While we can’t make you a meteorologist, we can certainly help you think like one. Quite understandably you’re not too concerned whether increased warm air advection will interact with a stationary front nearby, but you’re definitely focused on whether certain unexpected changes will disrupt your flight or cause difficulties in the terminal area. By working up from a basic understanding of atmospheric building blocks, we can help you get that sort of “master plan” understanding of the weather pattern and anticipate changes before they happen.
Crash Course 101
To get our forecast mojo in gear, let’s reduce things to the essentials. There’s no need for math equations or pages of physics. The thing that the atmosphere reacts to most is unequal distribution of heat. This sets air in motion and leads to the motion (advection) of air masses and the transport of humidity (moisture) from one place to another, like from ocean to land (the most prominent example being monsoon rains). It might seem that low pressure systems “cause” weather, but they’re in fact a reaction to an unstable distribution of heat in the atmosphere, in the same way that a tornado is the end result of horizontal shear being tilted into the vertical under a thunderstorm.
The most elemental cause of motion in the atmosphere is the uneven heating of the earth. The difference in temperature between the polar regions and the tropics drives a circulation called the Hadley cell, one of the largest and most dynamic circulations on earth. Heat rises in the tropics in the form of storm cells and thermals, and is usually sinking wherever there’s fair weather. But, more air rises overall than sinks.
Moving all this mass to the upper atmosphere results in a large high pressure area in the upper levels, located over the tropics. This air then flows north and south to the temperate latitudes. It eventually returns to the tropics as trade winds, the semipermanent northeast breeze that you feel when you’re in the Caribbean.
The air aloft over the tropics doesn’t flow all the way to the poles. Coriolis force begins acting on it and deflecting it to the right in the northern hemisphere. With more air coming in behind, mass “piles up” in this area like a large traffic jam. Barometers can sense this traffic jam aloft, as they’re a sort of scale that detects the mass of all the air between the ground and outer space. The barometer registers high pressure at the surface. This forms the semipermanent subtropical high, like the Bermuda and Pacific high, which extends all the way from the surface to the stratosphere.
Looking Up North
As you might guess, cold air from the poles is dense, hugging the surface, and flows away from the poles toward the temperate latitudes. It too turns to its right in the northern hemisphere, turning from a northerly to a northeast wind. This gives the concept of “polar easterlies.”
But in practice we don’t really see much of these polar easterlies, because the intense contrast between polar air and warm tropical air produces a sort of battleground, overwhelming any permanent circulations. The interface between cold air and warm air forms the polar front, which tends to ring the globe in the temperatre latitudes. The polar front is baroclinically unstable (unstable because of temperature contrasts), and the energy is released in the form of powerful frontal systems, the same ones we’re all so familiar with in the United States.
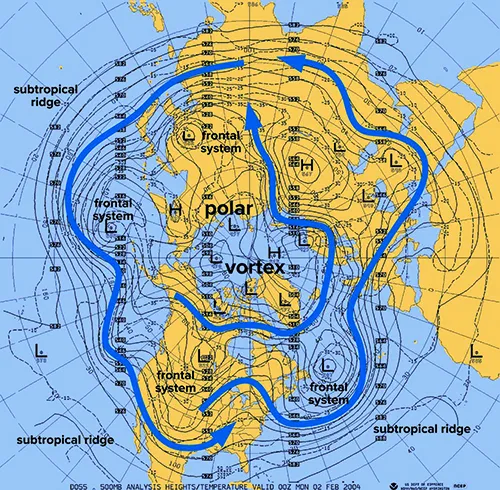
This all describes the global circulation. This is what powers most of the weather around the world. There can also be localized circulations superimposed on the global circulation. A good example is the semipermanent heat low located in the southwest United States during the warm season. It measures only hundreds of miles in size, influencing weather around the area. Mountain winds are another kind of localized circulation. Finally, weather is influenced by migratory weather systems such as frontal lows, troughs, and squall lines, which produce brief and temporary changes to the weather.
Then There’s the Jet Stream
One other concept that’s important is the relationship of temperature to pressure aloft. Warm air masses have weak density and great volume, extending their influence into the upper atmosphere in the form of high pressure. Forecasters use constant pressure charts, so these are also referred to as having “high geopotential height.” It’s sort of the same thing.
Likewise cold air masses have high density and low volume. This causes an observable pressure deficit in the middle and upper troposphere that intensifies with increasing height.
A good rule of thumb is that when there’s a cold air mass, there tends to be low pressure (low geopotential height) aloft. It intensifies with height. This often manifests as upper-level roughing. When there’s a warm air mass, there’s often high pressure (high geopotential height) aloft. It also intensifies with height. This is often seen as upper-level ridging. If you’re an amateur forecaster, this is a good concept to memorize.
Our first good example is the large mass of cold air covering the polar regions. It’s always cold up there. This means that we will usually find a large polar vortex. This isn’t the same polar vortex from news headlines in 2014, rather this refers to the massive low pressure area aloft that’s found literally all the time over the polar regions. Most of the time the northern polar vortex is loosely centered over northern Canada, but it occasionally shifts to the Arctic Ocean, Greenland, or northern Siberia.
When we have two air masses with different temperatures right next to each other, it presents a difference in upper-level pressure that intensifies with height. So we get a pressure gradient along the boundary (difference in pressure per unit of distance) that intensifies with height. This is what causes the jet stream. As you might imagine, the jet stream snakes around the polar vortex like water swirling in a toilet bowl.
The jet stream flows from west to east. Like most large-scale motion in the northern hemisphere, air seeks to flow so that low pressure is on its left side (looking in the direction of travel) and high pressure is on the right side. For the jet stream, this places the polar vortex on the left side and the subtropical high and equatorial high on the right side.
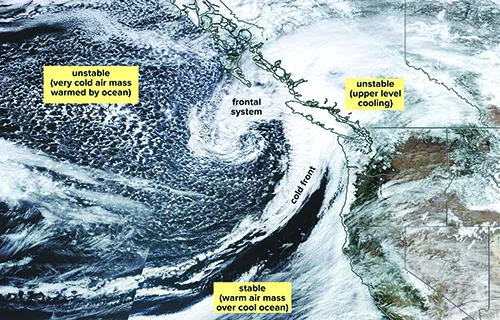
Finally, large scale wind flows are strong when the horizontal pressure gradient is strong. And for the upper levels, this means that the jet stream is strongest wherever low-level temperature contrasts are the strongest. It literally sits above the polar front, wherever it is. The polar jet and the polar front are synergestically linked and one follows the other wherever they go. An intensifying front means an intensifying jet. This is easy, right?
Applying To Aviation
Now we have enough information to make some guesses about the weather. Remember our example at the beginning? You’re in Kansas and winds at 25,000 feet will be from 220 at 80 knots.
We see that winds at 25,000 feet are flowing southwest to northeast. If we’re riding along with this wind, to our left will be low pressure and to the right high pressure. Since upper-level conditions are affected mostly by low-level temperature contrasts, we can immediately picture a cold air mass dominating the Rockies or Great Basin, while a subtropical ridge probably covers much of the southeastern states. Weather is likely to be better to the southeast if the front hasn’t passed already.
Also winds of 80 knots suggest a jet stream. This tells us that a frontal system is likely to be active in Kansas, or is approaching the area. It’s a time to be extra cautious and go over TAFs, area forecasts, and SIGMETs.
What about that 3000-foot ceiling? Many pilots will just take it at face value and look at the TAF to see when it clears up. But for a meteorologist, that ceiling suggests there’s a source of moisture advection or low-level lift. Which one is it?
Strong southerly low-level winds (at about 2000 to 5000 feet) usually indicate a low-level jet bringing up moisture from the Gulf, and this is a common source of clouds in spring and early summer, especially if surface winds are gusty. But it’s November and conditions are cool, so this should focus your attention on low-level lift.
Since the winds are out of the east and it’s the cool season, we likely have a warm front to the south, with a low pressure area somewhere to the southwest. Using some of the other concepts we’ve learned in these articles, we also know that warm fronts in an area of strong lift are commonly associated with mixed and clear icing. We’d better check on that and know our freezing levels before we open the flight plan.
Other Tips and Tricks
Those are just some examples. There’s a wealth of information in other details from ASOS reports. One of my favorite examples that isn’t used nearly enough is wind gusts. Wind constantly changes from second to second, and we can describe it in terms of lulls, peaks, and averages. Wind speed is the average speed during a 2-minute period (10 minutes outside the US).
Gusts are actually quite special. It officially exists when there’s a spread of 10 knots or more between the lull and the peak during the sampling period. This means when winds are reported to be 24 gusting to 32 knots, we can safely assume that the lulls are 22 knots or lower.
Meteorologically, this is important because gusts are a manifestation of turbulence and eddies in the atmosphere. High winds can generate eddies through frictional effects, as air passes over buildings and trees, and this is magnified by wind speed. But instability has a major impact, helping the formation of eddies and turbulence cascades.
This means when an ASOS reports a gust, this is a sign that there’s either high winds or high convective instability. Often it will be obvious which one it is. If winds are gusting to 67 knots, most of the time this is because thunderstorm outflow (or a strong hurricane) is passing through the area. On the other hand, a 27-knot gust in December in New York probably means convective instability. Convective instability is the result of a sharp temperature decrease with height, the so-called “cold over warm” situation.
In the summertime we see this quite often due to intense surface heating. It’s no surprise that on a hot day in June in Phoenix, we will see gusts and the occasional lazy dust devil swirling around the airfield. In winter, the biggest source of instability is air mass modification, when a very fresh, cold air mass is rapidly moving southward across relatively warm terrain. In the old Bergeron air mass scheme, these air masses were labeled “cPk” (continental polar cold) on weather maps. Visibility is excellent, winds are gusty, it’s cold, there’s lots of mechanical turbulence, and if there’s enough moisture there might be bands of blue sky alternating with patchy snow showers. This type of destabilization from the ground up tends to be somewhat shallow, often no more than 5000 to 10,000 feet deep, so most of your turbulence will be concentrated there.
If this doesn’t quite fit the pattern, maybe instead of warm air replacing the bottom of the atmosphere, cold air is replacing the top. This is very common in spring and fall, especially in the Great Plains, as powerful upper-level systems approach from the west. When you hear about weather being caused by an “upper level disturbance,” as often happens in California in March, or by a “short wave disturbance,” this is instability from
cooling at the top. These types of systems steepen atmospheric lapse rates through a deep column and destabilize the atmosphere. Because of this, turbulence can range through great heights, all the way to the stratosphere in some cases, producing clear air turbulence (CAT).
Those scenarios described above account for maybe 90 pecent of gusty wind reports. Next time you spot one in the METAR reports, try choosing what the cause is. If it’s summertime, though, this might be a bit too easy. Still though, if the gust is unusual, it points to something unusual that might be going on: perhaps more instability than expected, more turbulence, and enhanced precipitation potential.
Try It
This should give you most of the basics you need for picking apart the atmosphere. While our goal isn’t to make you a forecaster in three scant pages, these concepts will give you a good start for using the available data to fit the puzzle pieces together as a pilot to extract as much information as possible from the situation.
Good read thanks Tim! How about an article on identifying vort max on upper level charts?? And areas of upper level divergence. Please keep writing, yours are the only one I read.
Bill